What are Scintillation Materials?
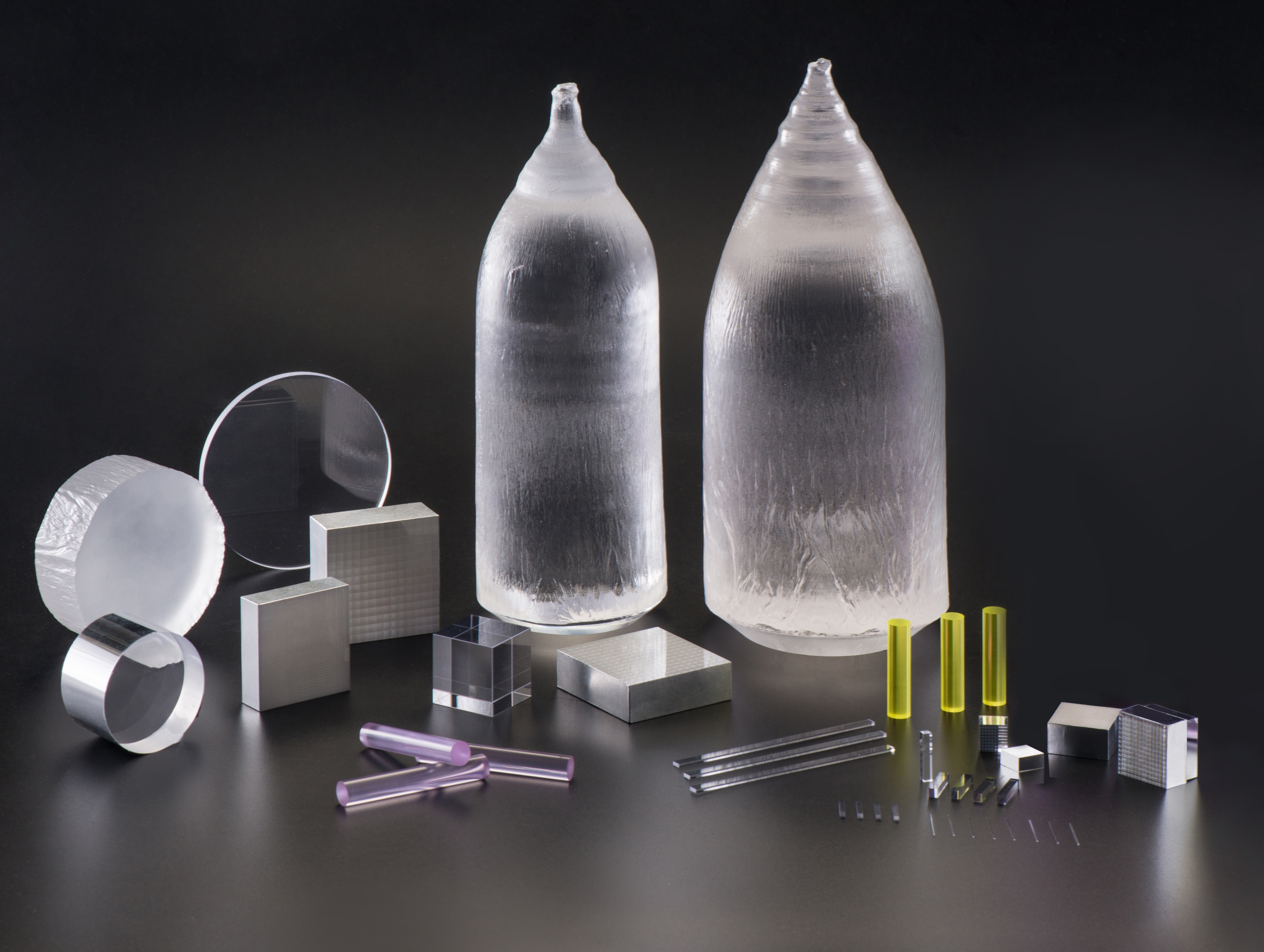
Definition and Application Principle
Scintillation materials are substances capable of converting high-energy radiation like X-rays or gamma rays into visible or near-visible light. Widely employed in medical diagnostics, high-energy physics, and geophysical exploration, scintillators come in various forms including gaseous, liquid, or solid compositions, and can be organic or inorganic such as glass, single crystals, or ceramics.
Detectors utilizing scintillators typically consist of two main components: the scintillator material itself and a photodetector, which could be either a photomultiplier tube (PMT) or a photodiode. The role of the photodetector is to transform the emitted light from the scintillator into an electrical signal for further analysis.
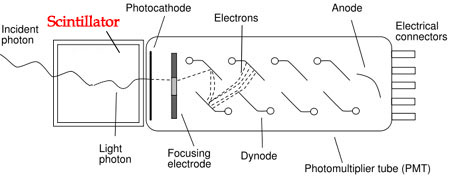
Figure 1: Schematic diagram of a scintillation detector comprising a scintillation material coupled to a photomultiplier tube.
Photomultiplier tubes, the most commonly used photodetectors, are constructed with a photocathode followed by a series of dynodes. When a photon strikes the photocathode, it emits a photoelectron, initiating a cascade of electron multiplication across the dynodes. The resulting amplified signal is then collected at the anode and transmitted to measurement circuits. The magnitude of the electrical signal is directly proportional to the number of photoelectrons generated.
The mechanism underlying scintillation involves three primary subprocesses: conversion, energy transfer, and luminescence. When high-energy radiation is absorbed by the scintillator material, it generates primary electron-hole pairs, which subsequently produce secondary pairs via a cascade effect. These excitations undergo thermalization, reaching the bottom of the conduction band and the top of the valence band within a picosecond. Subsequently, free electron-hole pairs migrate through the material, transferring energy to luminescent centers in a rapid process lasting between 10^-12 to 10^-8 seconds. Finally, luminescence occurs, with the duration dependent on the specific luminescent centers within the material.
The emitted photon's energy is a crucial parameter for distinguishing between different radioisotopes, as it correlates with the energy of the incident radiation. Favoring the photoelectric effect enhances accuracy, as this mechanism ensures the complete absorption of incoming radiation. Materials with high atomic number and photoelectric fraction are preferred to increase the probability of the photoelectric effect.
Scintillators exhibit several characteristics essential for their application, including light yield (photons/MeV), energy resolution, decay time, afterglow, and stopping power, which determine their suitability for various tasks.
Fabrication of Scintillators
Traditionally, inorganic scintillators are fabricated as single crystals using methods like the Czochralski or Bridgman-Stockbarger techniques. The Czochralski method involves slowly pulling a seed crystal from a melt, while the Bridgman-Stockbarger method lowers a sealed ampoule containing the molten material into a cooler region to facilitate crystal growth. Each method is tailored to specific materials based on factors such as melting point and sensitivity to environmental conditions.
The Czochralski method, depicted in Figure 2, entails attaching a seed crystal to the bottom of a vertical arm, just barely touching the surface of the melt. The arm is then gradually raised, allowing a crystal to form at the interface between the seed crystal and the melt. Typically, the crystal is rotated slowly to prevent inconsistencies in the liquid from transferring to the crystal. Controlled variations in the pulling rate, monitored by computer-controlled apparatuses, enable the production of crystals with desired diameters. This method is commonly utilized for materials with high melting points.
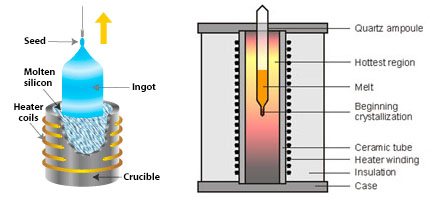
Figure 2. Czochralski apparatus (left)
Figure 3. Bridgman-Stockbarger furnace (right).
In contrast, the Bridgman-Stockbarger method, illustrated in Figure 3, involves heating a polycrystalline material within a sealed ampoule, shaped cylindrically with a conical lower end. The molten material is maintained by heaters within the ampoule. As the ampoule is gradually lowered into a cooler region, a crystal begins to grow from the conical tip. The rate of descent matches the growth of the crystal, ensuring that the temperature at the interface between the crystal and the melt remains constant. Successful execution of this method results in the entire molten material within the ampoule crystallizing into a single large crystal. Impurities accumulate at the interface between the melt and the solid as it moves upward, ultimately concentrating in the upper part of the crystal. This method is particularly suitable for materials with lower melting points and those sensitive to atmospheric conditions, such as Strontium Iodide.
Recent R&D Progress of Scintillators
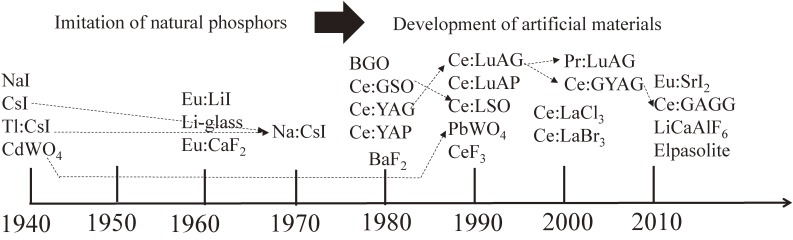
Figure 4. R&D History of common scintillators. Arrows indicate modifications of materials.
Initially, alkali halides (e.g., NaI and CsI) and natural phosphors (e.g., CaF2 and CdWO4) garnered attention for investigation. Notably, CaF2 and CdWO4, known as fluorite and scheelite in mineralogy respectively, emerged as early candidates. The period from the 1940s to the 1960s witnessed the development of foundational scintillator materials, including Tl-doped NaI, Tl-doped CsI, and Eu-doped CaF2.
Subsequently, in the 1980s, researchers pioneered the development of novel materials, such as Bi4Ge3O12 (BGO), which exhibited exceptionally high effective atomic numbers. BGO found applications in early positron emission tomography (PET) systems due to its favorable properties. The advent of BGO spurred significant research and development efforts, notably the incorporation of Ce-doping into insulating host materials, a technique that has since become standard practice in scintillator development. Ce-doped Lu2SiO5 (LSO) and its yttrium-admixed counterpart, LYSO, have emerged as standard materials, supplanting BGO in modern PET systems.
.jpg)
Figure 5.LYSO (Ce)
Recent innovations include the development of Ce-doped Gd3(Al,Ga)5O12 (GAGG), which has garnered widespread use alongside Si-based photodetectors. Notably, Ce-doped garnet scintillators offer unique emission wavelengths, making them suitable for photodiode-based radiation detectors. Figure 4 provides a comprehensive overview of the research and development trajectory of common scintillator materials.
Figure 6. GAGG(Ge)
What does Shalom EO Provide?
Shalom EO is a leading provider of scintillator materials, offering a wide range of options to suit various applications. Their scintillator materials primarily fall into two main categories: crystals and plastics.
Crystal scintillators are composed of inorganic crystalline materials, often doped with specific impurities to enhance their scintillation properties. These crystals typically offer high light output efficiency and excellent energy resolution, making them suitable for precision measurements in fields such as particle physics, medical imaging, and radiation detection. Examples of crystal scintillator materials include sodium iodide doped with thallium (NaI(Tl)), cesium iodide doped with thallium (CsI(Tl)), and lutetium yttrium orthosilicate (LYSO). These materials are widely used in applications requiring high performance and accuracy.
Plastic scintillators are composed of organic polymers doped with fluorescent dyes or other scintillating agents. These materials offer versatility, ease of fabrication, and cost-effectiveness compared to crystal scintillators. Plastic scintillators are commonly used in applications where flexibility, durability, and low cost are important factors, such as radiation monitoring, nuclear safeguards, and industrial process control. These materials provide efficient detection of ionizing radiation while being customizable for specific requirements.
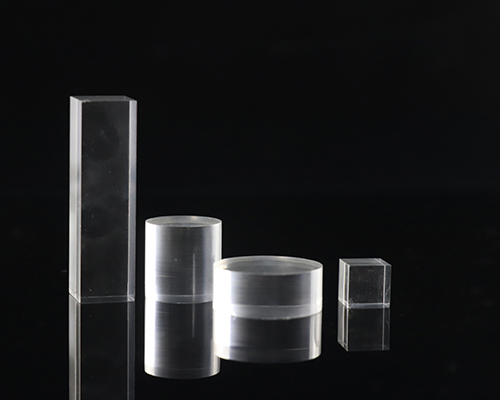
Figure 6. Plastic Scintillation material
Hangzhou Shalom EO offers various inorganic scintillators, like CsI(Tl), LYSO(Ce), CsI(Na), BGO, YAG(Ce), CaF2(Eu), BaF2, CdWO4, GAGG(Ce), LuAg(Ce), GOS, LSO, LaBr3(Ce), LaCl3(Ce) and CeBr3. which include polished crystals with or without reflectors, encapsulated crystals, pixelated scintillators, scintillator screens, and detector assemblies consisting of PMT, electronics, and scintillators.
Tags:
What are scintillation materials